Current Issue
Design of Biogas Digester for Small Community of Salalah Countryside, Oman to Support the Lighting and Cooking Electrical Load
Omar Al Gharibi, Dharmasa Pawar
Department of Electrical and Communication Engineering, College of Engineering Campus (Al Hail), National University of Science and Technology, Muscat, Sultanate of Oman.
--------------------------------------------------------------------------------------------------------------------
ABSTRACTBio-gas is a renewable energy source derived from the anaerobic digestion of organic materials such as agricultural, manure, and food waste. It emits methane and carbon dioxide, which reduce greenhouse gas emissions and promote circular economies by converting organic matter into valuable energy and nutrients. In this study, the biogas-based power generation possibilities are studied and reported based on the availability of biomass from animals like camels, sheep, goats, and cows. The data collection and analysis are carried out under different temperature conditions. The energy required is planned for eight people in each family. In the proposal work to design a digester, the location was chosen at the south-eastern part of the Arabian Peninsula (south of the Sultanate of Oman) for the place Madinat al Haqq. Afterwards, calculations are reported to estimate the size of the biogas digester and the output power generated from the digester. Here, the digester output is used for cooking and lighting for all members of the family. Further, the power output is viable based on the availability of biomass per day. The energy availability investigation is performed using the standard calorific value of the biomass per cubic meter. The proposed concept will allow the remote community to use a portal-sized biogas digester for their necessary electrical home load. Furthermore, the selection of the burner is reported to utilize the biogas efficiently.
Keywords:Bio-gas, renewable energy, methane, carbon dioxide, Biomass, Digester, Calorific Value, Community, Anaerobic, standard calorific value.
1. INTRODUCTIONEnergy is crucial for fostering sustainable development across economic, social, and environmental dimensions. It powers industries, supports livelihoods, and influences environmental outcomes. Sustainable energy practices aim to meet present needs without compromising the ability of future generations to meet their own needs. Currently, fossil fuels like Oil, Coal and natural gas dominate the global energy landscape, fulfilling the majority of the world's energy requirements. However, their combustion releases greenhouse gases (GHG)[1], such as carbon dioxide (CO2) and methane (CH4), into the atmosphere, contributing to global warming and climate change. Biofuels, derived from organic materials such as crops, agricultural residues, or waste, have emerged as an alternative to traditional fossil fuels. They offer a renewable and potentially sustainable energy source, as the organic materials used in their production can be replenished through agricultural practices or waste management. Biofuels are particularly transformative for areas of high dependence on fossil fuels, like transportation, shipping, and aviation. This dependence will be lessened, and the burden on the environment concerning emissions that would have been occasioned through these modes of transport will be significantly reduced. Though biofuels initially faced a challenge with production costs, technological advancement and developed economies of scale are steadily driving down these costs. This form of projection anticipates that in 2045, biofuel production will be able to economically compete within a price bracket of $0.60 to $1.10 per Liter. One of the most immediate benefits that biofuels provide to the environment is the fact that they can drastically reduce GHGs relative to traditional fossil fuels. It has been indicated that biofuels will be able to reduce emissions by 60-95% relative depending upon the specific biofuel and how it is produced. Biomass resource modelling and bioenergy potential modelled for each country in the Gulf Cooperation Council significantly increase the plausibility and insight of the adoption of biofuels within the region. Table 1 displays the main results of the modelling study with the biomass resource and bioenergy potential in each GCC country. Such analyses are based on estimating biomass availability, technological capabilities, and environmental considerations for projecting the potential contribution of biofuels in meeting regional energy systems and greenhouse gas emissions targets. Biofuels present a promising avenue to fulfil sustainable energy goals with dual economic, environmental, and social benefits. Their continued development and adoption are integral to transitioning towards a more sustainable energy future.
Table 1 Biomass potential in GCC region [2]
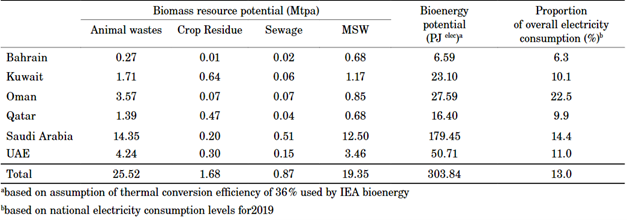
According to the data from Table 1, Oman has a high potential of biomass resource that can be converted into a large scale of energy. Nowadays, (in application of the vision of Oman 2040), Be'ah is also planning to develop a structure to process 4,000 tons of MSW per day, or more than 60% of the MSW that is currently landfilled and produce at least 140 Megawatts per day of electricity that could be connected to the national grid [3]. So, this will decrease the need for oil, fossil fuels and nuclear fuels. Then, gas emission such as carbon dioxide (〖CO〗^2), nitrous oxide (N_2 O) and methane (〖CH〗_4) from burning and harmful animal landfills are also decreased. That is because livestock is contributing to the rise of gas emission. In this paper, our focus is on the south part of Sultanate of Oman. The place is famous for livestock such as cows and camels etc. According to the research by [4], Dhofari cattle breed forms a major part of the total number of Sultanate of Oman. The aim of this paper is to design a biogas digester plant. So, primarily the cows’ waste will be collected and then processed by the digester. After that it will be converted to biogas. The outcome from this process helps local people to get electricity for cooking and lighting. The overall practice is accomplished by low energy consumption. In addition, it is simple to use and has low operating costs.
2. PROPOSED METHODOLOGYBiomasses are of three categories:
i. Solid mass like (wood and farms residue) that can be burned and directly converted to heat energy.
ii. Biomass is converted into ethanol or methanol as a liquid fuel.
iii. Biomass is processed (fermentation or anaerobically) to produce biogas.
Previous research reported that wet biomass contains (50-90 %) water. Then this type of biomass is not economically to be transported for long distances. So, the biogas plant should be close to the source. In this proposal, the biogas digester will be positioned at Latitude 54° 57' 0"; Longitude 17° 37' 26", with elevation of 1756 m for the place Madinat al Haqq, Figure 1 Here the required digester is designed for the plant, by considering 0.227 m3 of biogas for cooking and 0.126 m3 of biogas for lighting in day for each member of the 5 families of 8 members. As per [5] said, 2 m^3 of digester and 45 kg of availability of fresh cow’s manure per day is much suitable for cook a day’s meal for small family. Moreover, 1 kg of cow’s sludge can produce about 0.2-0.4 m^3 of gas [5]. A normal mixture of wet dung contains about 7-9% of solid. For that, the ratio of wet dung to water is 1:4. So, 1 kg of water is to be added to the mixture to form the slurry. For example, cows dung contains 0.186 m^3 of gas given by 1 kg/day/cow [5]. According to the enumeration from the ministry of agriculture, fisheries, and water resources, the total number of livestock is dramatically increasing over the years as shown by Table 2 Table 2below [6].
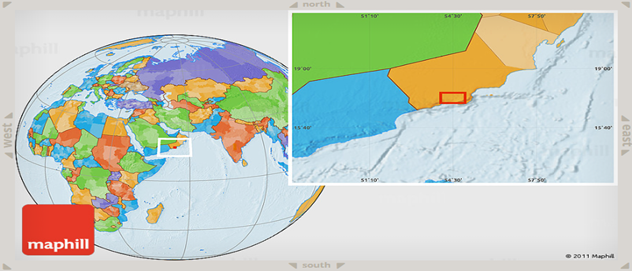
Figure 1 The location of Madinat al Haqq, Sultanate of Oman [7].
Table 2 Evolution of the number of economic animals in Oman [6]
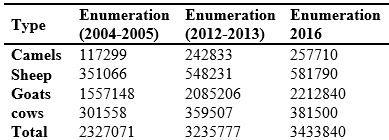
The largest part of the Sultanate’s livestock is concentrated in the Dhofar governorate, where the total number of livestock in the governorate according to the agriculture enumeration for the year 2016 reached 170 thousand of camels, 243 thousand of cows [8]. The process takes place at an annual low temperature 22.17ºC (71.91ºF) and annual high temperature 30.57ºC (87.03ºF). In this study, an anaerobic digestion method will be used as wet biomass conversion. Anaerobic digestion is the process that uses microorganisms like bacteria to produce biogas. The general equation for the process is like Equation (1):

For cellulose this become as Equation (2):

The gas production is about (450-500) litter per kg. In practice 0.2-0.4 m^3 of gas is produced per kg of cow’s dry digestible manure. The output premium gas produced is methane (〖CH〗_4), carbon dioxide gases (〖CO〗^2) that need further treatment and minimum impurities such as hydrogen sulfide (H_2 S). The output gas can be burned directly, or it can be modified to a synthetic gas after removal of (〖CO〗_2) and impurities. Then, the throughput of 5 kg of slurry will be per m^3 of liquid. The residue is rich in protein, so it can be used as animal feed after it is dried. Or a slurry that can be directly utilized by plants [5].
A. Some factors affecting the digestion process:
PH or hydrogen ion concentration: pH should be maintained between 6.5-7.5 to maintain a constant supply of gas.
-
Temperature:Temperature: the gas generation is started from 20° C and stopped at 10° C. However, the bacteria work best at value is between 35°-38° C.
-
Loading rate: practically 1.2-5.3 kg is suitable for the digestion process. Moreover, if the digester is overloaded, there is a chance to stop the fermentation by accumulated acid.
Carbon to Nitrogen Ratio:C/N ratio should be maintained to the best value which is 30 to get best digestion of the input material. Otherwise, too much carbon leads to stop the process and too much nitrogen will inhibit the bacteria and stop the fermentation.
-
Retention time:the time to keep the manure inside the digester. For cow dung it varies because of temperature and other factors but it may take from 30 to 60 days in some regions.
B. Biogas plant design
The size of the digester depends on the required biogas for human lighting and cooking use. Although, for producing biogas from cow manure, the fixed dome digester design is preferred due to its lower production costs and longer lifespan [9] as shown by Figure 2. So, a cylindrical shape digester was chosen. Figure 3 shows the schematic and experimental diagram of the digester according to Sharma A [10]. Bricks and cement can be used for constructing the plant with some other materials. Most of the construction will be undergrounded to maintain the temperature value to be constant. According to Rai [5], Gas usage for cooking and lighting per person per day is 0.227 m3 and 0.126 m3 /person/day alternately. The biogas digester is thus mathematically planned to generate a total of 14.12 m3/day of biogas for eight people of the five families considered in this study. The size of the biogas digester was determined by considering the following factors:
- The number of cows (50),
- The retention time (tr) e.g., 30 days for cows.
- The temperature (300C)
- Dry matter consumed is 2kg/day/cow.
- Biogas yield (C) is 0.24 m3 per kg.
- The efficiency of the burner (μ) is 60 %.
- Methane proportion is 0.8.
- Heat consumption of methane (Hm)=28 MJ/m3
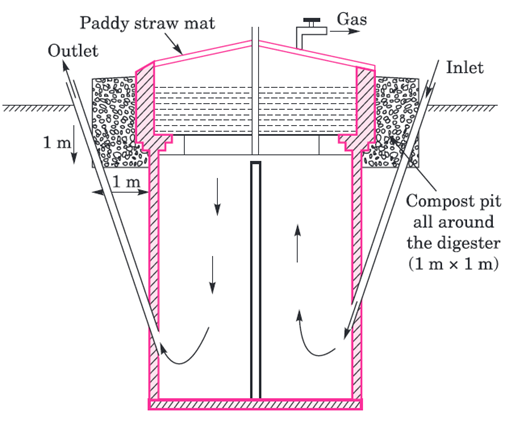
Figure 2 The design of biogas digester [5]
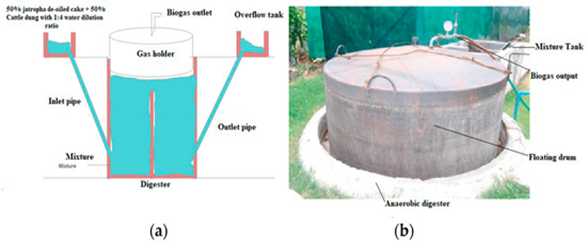
Figure 3 The schematic and experimental diagram of the digester [9]
3. DESIGN CALCULATIONS FOR DIGESTER:
The mass of the dry input can be calculated by using Equation (3):

The volume of the fluid in the digester is calculated by using Equation (4):

*where ρm is the density of the dry material in the fluid= ~ 50 kg/m3
The slurry volume is calculated by using Equation (5):

The weight of the daily biomass can be calculated by using Equation (6):

*where C is the gas production rate
0.186 is the amount of gas in m3 given by 1 kg of cow’s dung.
The volume of the digester is calculated by using Equation (7):

*where tr is the retention time in the digester= ~ 30-60 days
The volume of the biogas can be calculated by using Equation (8):

The power available from the digester can be calculated by using Equation (9):

*Where
μ is the efficiency of the burner =0.6 ,
Hm is the heat consumption of methane= 28 MJ/m3,
Fm is the fraction of methane in biogas= ~ 0.7
Vb is the volume of the biogas=48 m3/day
The shape of the digester is chosen to be cylindrical. The Hight and diameter of the digester can be found by the Equation (10):

The digester diameter can be calculated by using Equation (11):

The digester height can be calculated by using Equation (12):

Where,
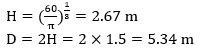
4. BIOGAS BURNER DESIGN
Biogas is consisting of 50-70% methane (CH4) and 25-40% carbon dioxide (CO2), it may also contain (1-5%) other gases such as the hydrogen sulphur (H2S), ammoniac, water vapour and volatile organic compounds. To guarantee the effectiveness, reliability, and safety of gas-fired equipment, the gas supply to the cooker must be delivered properly. 1.46 mm was the jet diameter that was found for biogas. The Wobbe Index could be improved by the interchangeability of biogases with other gases like propane or butane. “The Wobbe Index (defined as higher calorific value / square root of relative density) is a key indicator of the interchangeability of fuel gases” [11]. CO2 must be eliminated to adjust the calorific value. 10 mbar is the ideal supply pressure for biodigester biogas. According to the research, the burner's design, and the pre-mixing procedure, which involves mixing biogas with primary air, results in a more uniform mixture of fuel and oxygen. 49.44% were found using biogas with a calorific value of 22 MJ/m3 under accurately controlled conditions [11].
5. RESULTS AND DISCUSSIONS
In this study, the formulated scenario is planned in accordance with some assumptions. The digester will be fixed under the ground to maintain the heat value at (300C). The gas production rate of cow’s manure was calculated to 0.24 m3/day. 14.12 m3/day of biogas is required to be produced by the digester. Therefore, almost 59 Kg/day is required to feed the digester. The result shows that 100 Kg/day can give 24 m3/day of biogas. Therefore, 41% of biogas will be available to be consumed. The digester design is set to be more economical and cost durable and easy to maintain. Moreover, the fixed dome model digester will be the best choice for the low- and medium-income local people which live in rural areas. That is because normally these digesters have been made of mild steel or clay. Moreover, Biogas stove designs are specifically developed for the low-pressure gas burners. So domestic cookers that typically use natural gas (NG) or liquefied petroleum gas (LPG) can burn biogases, but the jet diameter needs to be adjusted correctly.
6. CONCLUSIONS
This study presents a construction of cylindrical shaped anaerobic biogas digester for small communities of “Madinat al Haqq” for house usage. It is expected that the study will make it easy to understand the design process as well as the mathematical calculations. The case study is carried out on five families for which each family holds eight members. The digester is designed to produce 24 m3/day of gas. The dimension of the digester is given like 2.67 m in height and 5.34 m in width. To build the bricks and cement are used for the construction of the plant. This construction will be affordable for such communities considering the low investment cost. Further feasibility and construction cost study is recommended. Furthermore, intensive research is needed for biogas production and storage and efficient utilization without leakage. Results in this previous research vary because a given domestic cooker's efficiency is not consistent due to changes in the environment and the type of composite fuel used. Moreover. Because H2S burns, more research needs to be done on the corrosion resistance of burner crowns and pan supports.
REFERENCES
- Y. F. Lim et al., ‘Review of biowastes to energy in Malaysia: Current technology, scalability and socioeconomic analysis’, Clean Eng Technol, vol. 4, Oct. 2021, doi: 10.1016/j.clet.2021.100257.
- F. O. Mahroogi et al., ‘A critical review of biofuels as an alternative fuel to diesel in gulf regional corporation (gcc) region: Current market trends and future opportunitie’, Communications - Scientific Letters of the University of Žilina, vol. 24, no. 1. 2022. doi: 10.26552/com.C.2022.1.B67-B73.
- Z. A. Abdulkhaliq, ‘be’ah-Waste Management in the Environmental Age’.
- S. Bahashwan, ‘The Dhofari cattle breed; productive and reproductive performance’, Livestock Research for Rural Development, vol. 32, no. 2, 2020.
- G. D. Rai, ‘NON-CONVENTIONAL SOURCES OF ENERGY [A Textbook for Engineering Students]’, 1979. [Online]. Available: www.khannapublishers.in
- ‘Livestock’. https://www.maf.gov.om/Ministry/dynamicPage/3119 (accessed Apr. 13, 2023).
- ‘Maphill: Web’s Largest Map Gallery’. http://www.maphill.com/ (accessed Apr. 13, 2023).
- Saleh, ‘Oman News Agency’, 2022. https://omannews.gov.om/topics/ar/116/show/407646 (accessed Apr. 13, 2023).
- N. Sawyerr, C. Trois, T. S. Workneh, O. Oyebode, and O. M. Babatunde, ‘Design of a household biogas digester using co-digested cassava, vegetable and fruit waste’, Energy Reports, vol. 6, pp. 1476–1482, Dec. 2020, doi: 10.1016/j.egyr.2020.10.067.
- A. K. Sharma, P. K. Sahoo, M. Mukherjee, and A. Patel, ‘Assessment of Sustainable Biogas Production from Co-Digestion of Jatropha De-Oiled Cake and Cattle Dung Using Floating Drum Type Digester under Psychrophilic and Mesophilic Conditions’, Clean Technologies, vol. 4, no. 2, pp. 529–541, Jun. 2022, doi: 10.3390/cleantechnol4020032.
- C. Grima-Olmedo, A. Ramírez-Gómez, and R. Alcalde-Cartagena, ‘Energetic performance of landfill and digester biogas in a domestic cooker’, Appl Energy, vol. 134, pp. 301–308, Dec. 2014, doi: 10.1016/j.apenergy.2014.08.032.